Cosmic compact objects
Are the most extreme objects in the Universe, accumulating enormous amount of mass in a small space. They are formed as e result of supernovae explosions of the largest stars. The explosion is so powerful that the core of the star collapses inward, creating a black hole or neutron star. Neutron stars are made of atomic nuclei so crushed that this kind of matter cannot be obtained in Earth laboratories. On the other hand, black holes are regions of space-time where the compressed elementary particles are no longer able to create any gravitationally stable formation defined by the known laws of matter. Therefore, black holes are constantly collapsing stars, interacting with their surroundings only through the enormous force of gravity. Dead stars roam the cosmos. If on their way, they encounter areas of gas, they naturally initiate the phenomenon of accretion - i.e. the gravitational fall of gas onto a compact object.
Accretion
Of gas onto compact object, either onto black hole or neutron star, is one of the most powerful way of energy production in the Universe, which is than converted into electromagnetic flux. Therefore, astrophysicists, aiming to understand the nature of compact objects of various masses, they constantly monitor the radiation of matter, which disappears on our eyes, feeding black holes.
Therefore, there is a great importance to find common mechanism responsible for X-ray fingerprints of accreting compact objects – those with huge BH mass as active galactic nuclei (AGN) and those with stellar mass black holes or neutron stars. Over many years of X-ray observations we can formulate characteristic features, which are as fingerprints of accreting compact object.
Fingerprints
- DOUBLE PEAK CONTINUUM EMISSION
- SOFT X-RAY EXCESS
- IRON KΑ EMISSION LINE
- NARROW ABSORPTION LINES FROM IONIZED GAS
- SOFT X-RAY LAG
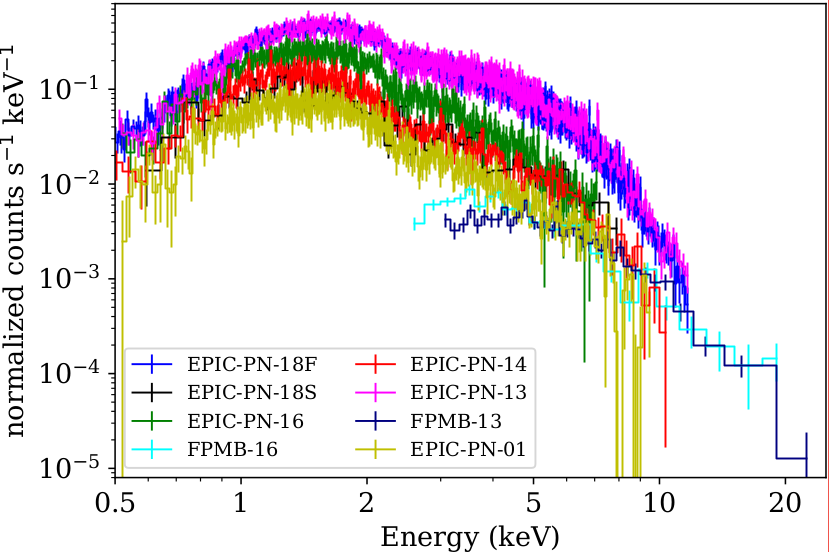
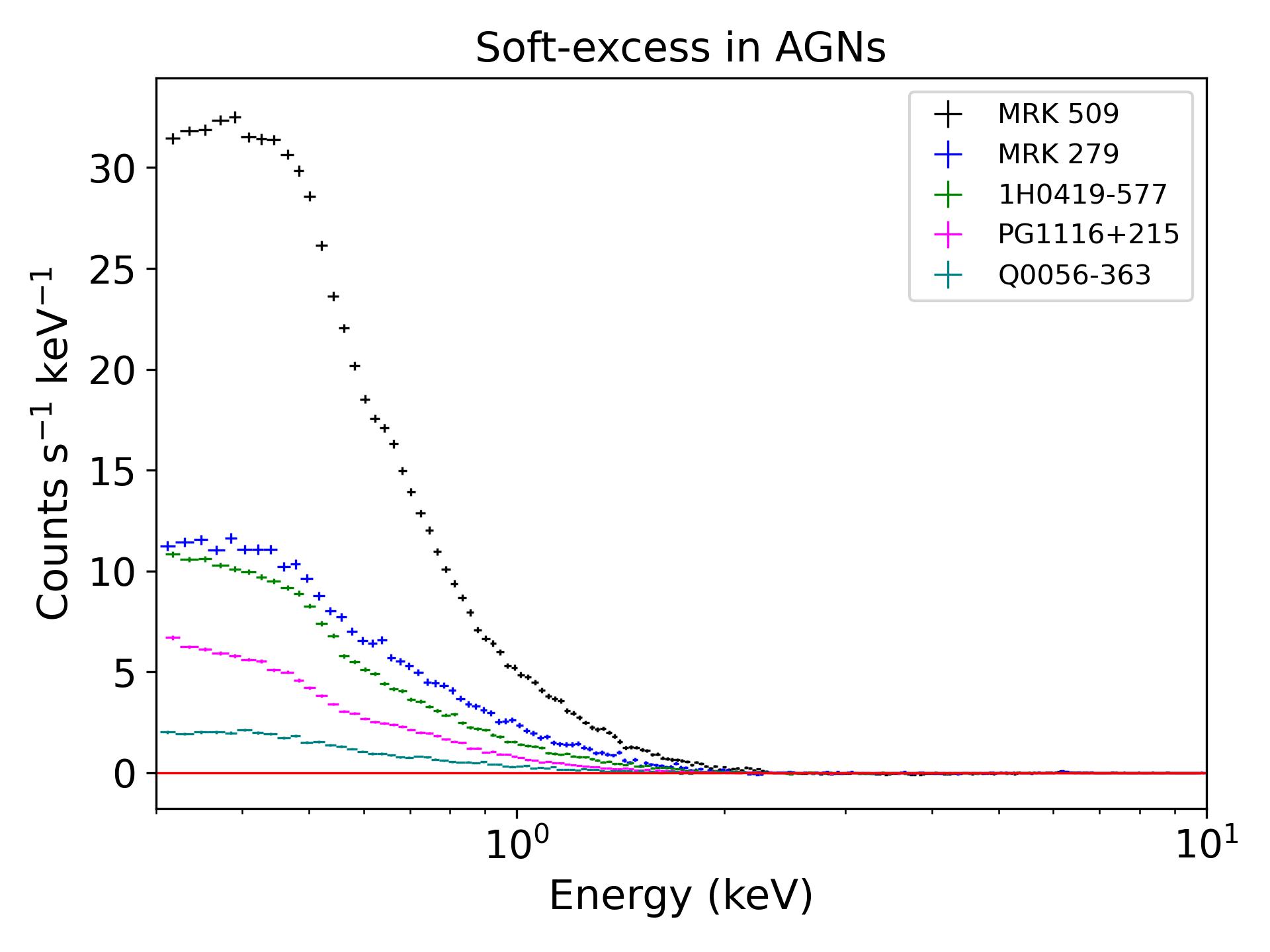
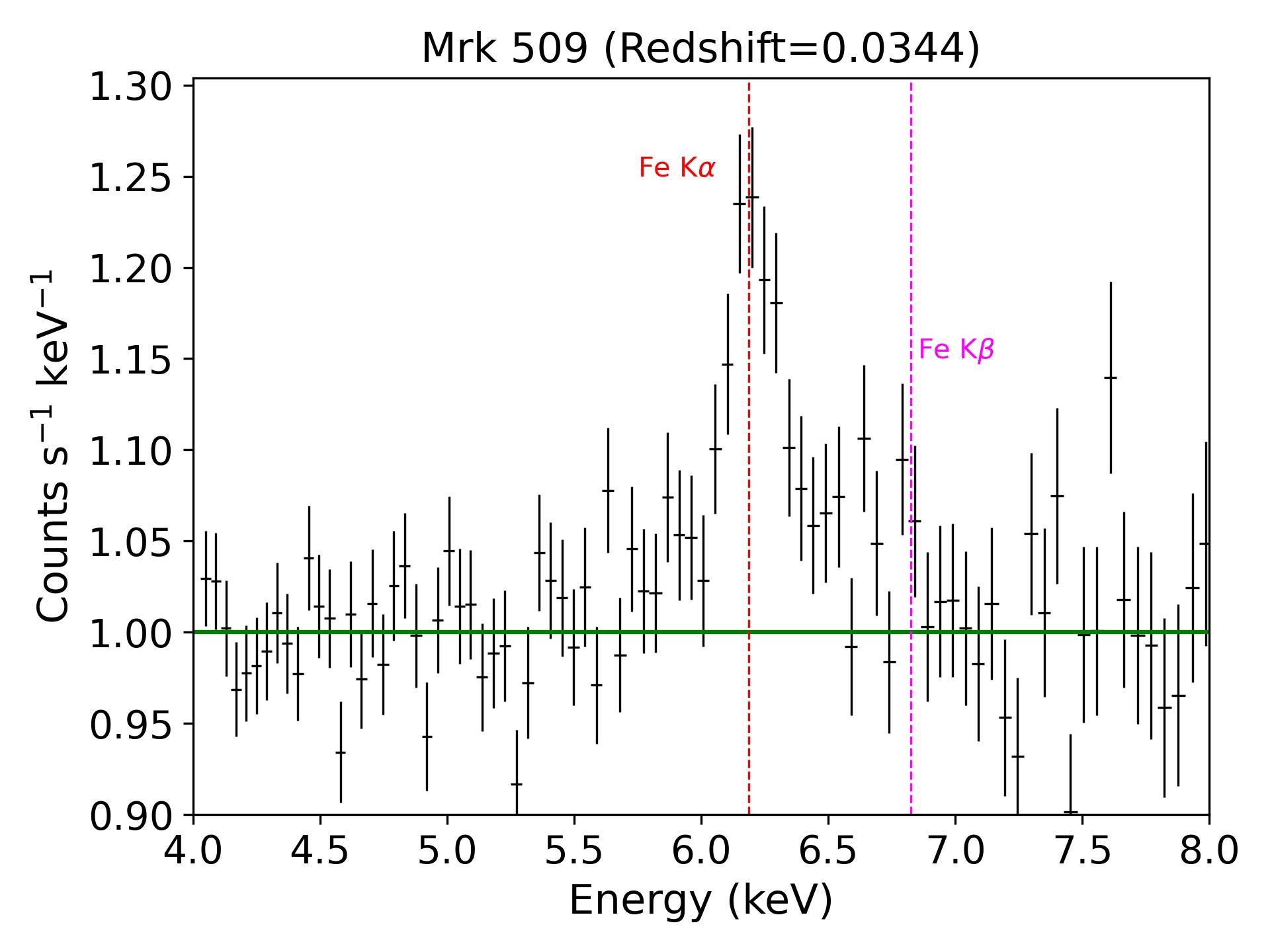
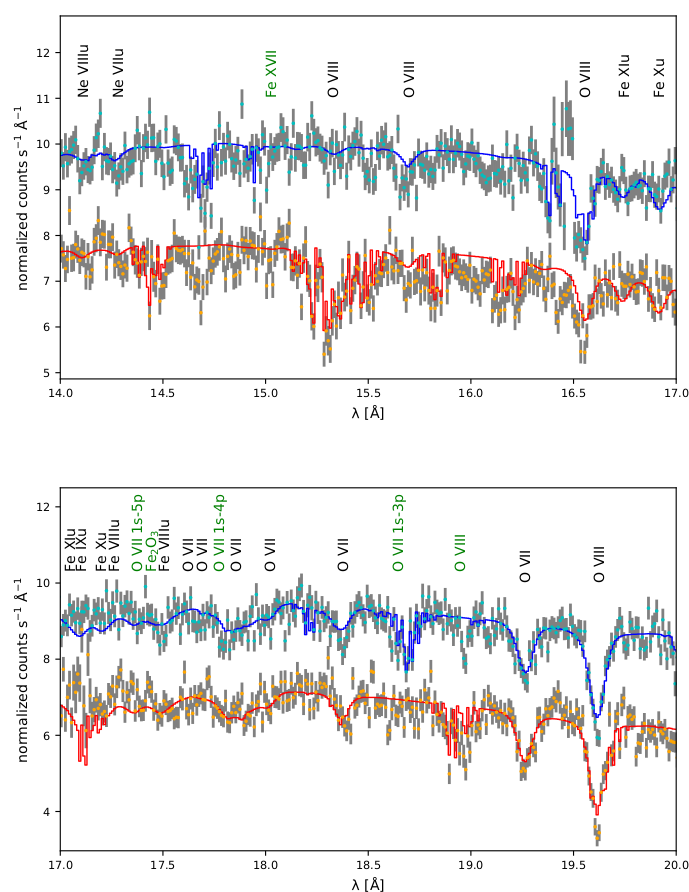
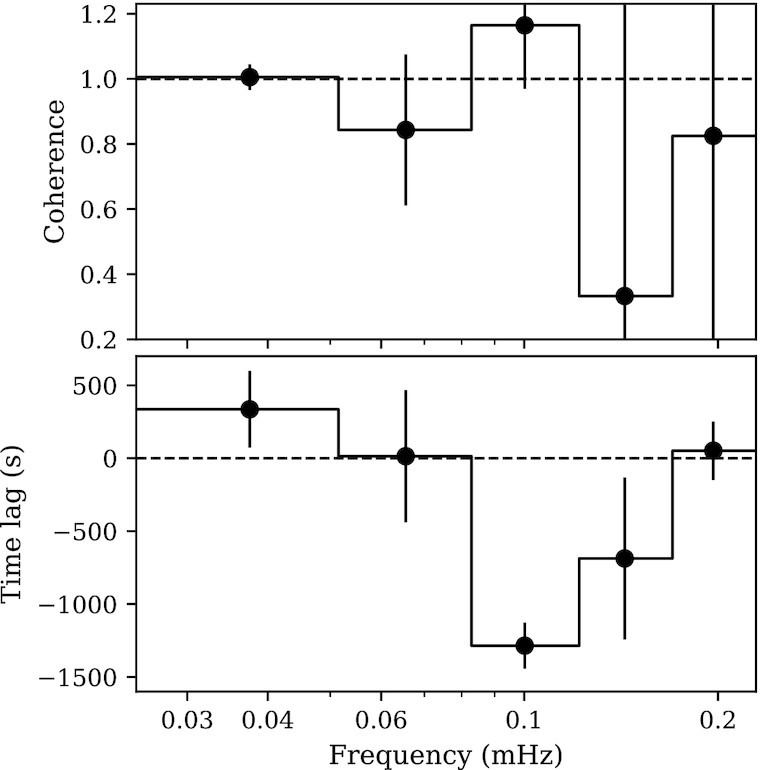
We explore
All these fingerprints in the context of developing new codes for computing advanced theoretical models of emission, collecting and analyzing X-ray data from currently working satellites, and finally making predictions of signal detected by future X-ray missions as ATHENA (European Space Agency, ESA mission) and ARCUS (NASA mission).